Sawbones biomechanical Test materials have become a reliable industry standard with over 20 years of research and verification
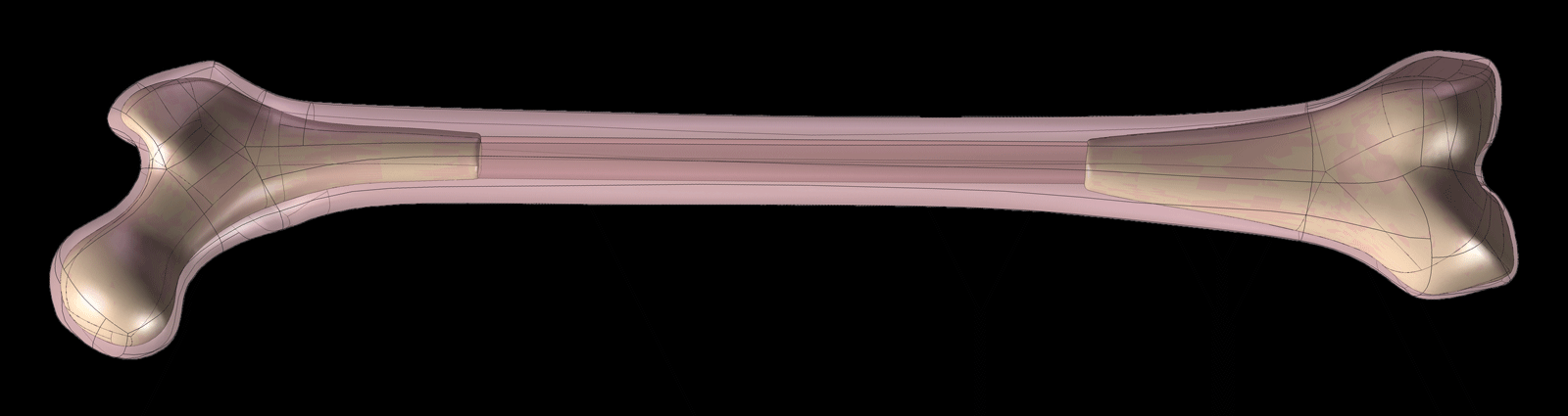
Biomechanical Validation Studies
Rigid Polyurethane Foam
ASTM F1839: Standard Specification for Rigid Polyurethane Foam for Use as a Standard Material for Testing Orthopaedic Devices and Instruments
Solid foam: 5, 10, 12, 15, 20, 25, 30, 35, 40, 50 PCF
Nagaraja S, Palepu V. Comparisons of Anterior Plate Screw Pullout Strength Between Polyurethane Foams and Thoracolumbar Cadaveric Vertebrae. J Biomech Eng. 2016;138(10). doi: 10.1115/1.4034427. PubMed PMID: 27536905.
Solid foam: 5, 8, 10, 15, 20 PCF
Laminated: 5PCF solid foam + 0.5mm short fiber filled epoxy
Open cell foam: 7.5, 15, 30 PCF
Calvert KL, Trumble KP, Webster TJ, Kirkpatrick LA. Characterization of commercial rigid polyurethane foams used as bone analogs for implant testing. J Mater Sci Mater Med. 2010;21(5):1453-61. doi: 10.1007/s10856-010-4024-6. PubMed PMID: 20162325.
Solid foam: 12, 15, 20, 25, 40 PCF
Hsu JT, Huang HL, Chang CH, Tsai MT, Hung WC, Fuh LJ. Relationship of three-dimensional bone-to-implant contact to primary implant stability and peri-implant bone strain in immediate loading: microcomputed tomographic and in vitro analyses. The International journal of oral & maxillofacial implants. 2013;28(2):367-74. doi: 10.11607/jomi.2407. PubMed PMID: 23527336.
10 pcf solid foam
10 pcf solid foam + 1mm short fiber filled epoxy
10 pcf solid foam + 2mm short fiber filled epoxy
10 PCF solid foam + 3mm short fiber filled epoxy
7.5 PCF cellular foam + 2mm short fiber filled epoxy
10 PCF cellular foam + 2mm short fiber filled epoxy
12.5 PCF cellular foam + 2mm short fiber filled epoxy
20 PCF cellular foam + 2mm short fiber filled epoxy
Patel PS, Shepherd DE, Hukins DW. Compressive properties of commercially available polyurethane foams as mechanical models for osteoporotic human cancellous bone. BMC Musculoskelet Disord. 2008;9:137. PubMed PMID: 18844988.
Solid foam: 10, 20 PCF
Open cell foam: 5.5 PCF
Szivek JA, Thomas M, Benjamin JB. Characterization of a synthetic foam as a model for human cancellous bone. J Appl Biomater. 1993;4(3):269-72. PubMed PMID: 10146310.
Solid and cellular foam: 10 – 15 PCF
Szivek JA, Thompson JD, Benjamin JB. Characterization of three formulations of a synthetic foam as models for a range of human cancellous bone types. J Appl Biomater. 1995;6(2):125-8. PubMed PMID: 7640439.
Solid foam 15 PCF
Thompson JD, Benjamin JB, Szivek JA. Pullout strengths of cannulated and noncannulated cancellous bone screws. Clin Orthop Relat Res. 1997(341):241-9. PubMed PMID: 9269180.
Solid and cellular foam 10-20 PCF
Thompson MS, McCarthy ID, Lidgren L, Ryd L. Compressive and shear properties of commercially available polyurethane foams. J Biomech Eng. 2003;125(5):732-4. PubMed PMID: 14618933.
Cellular foam 7.5, 10, 12.5, 20 PCF
Short Fiber Filled Epoxy
Chong AC, et al., Fracture toughness and fatigue crack propagation rate of short fiber reinforced epoxy composites for analogue cortical bone. J Biomech Eng 2007;129(4):487-93.
Femur
Chong, A.C., et al., Fatigue performance of composite analogue femur constructs under high activity loading. Ann Biomed Eng, 2007. 35(7): p. 1196-205.
3406
Heiner, A.D., Structural properties of fourth-generation composite femurs and tibias. J Biomech, 2008. 41(15): p. 3282-4.
3403, 3401
Gardner, M.P., et al., Mechanical evaluation of large-size fourth-generation composite femur and tibia models. Ann Biomed Eng, 2010. 38(3): p. 613-20.
3406, 3402
Zdero R, et al., The effect of cortex thickness on intact femur biomechanics: a comparison of finite element analysis with synthetic femurs. Proc Inst Mech Eng [H] J. Engineering in Medicine, 2009. 224: p. 831-840.
3403
Zdero R, S.S., M Mosli, EH Schemitsch, The effect of load application rate on the biomechanics of synthetic femurs. Proc Inst Mech Eng [H] J. Engineering in Medicine, 2009. 224: p. 599-605.
3403
Zdero, R., et al., Cortical screw purchase in synthetic and human femurs. J Biomech Eng, 2009. 131(9): p. 094503.
3406
Zdero R, O.M., Bougherara H,Schemitsch EH, Cancellous bone screw purchase: a comparison of synthetic femurs, human femurs, and finited element analysis. Proc. IMechE: J. Engineering in Medicine, 2008. Vol 222(Part H): p. 1175-1183.
3406, 3406-4
Nicayenzi, B, S Shah, E H Schemitsch, H Bougherara, and R Zdero. The biomechanical effect of changes in cancellous bone density on synthetic femur behaviour. Proceedings of the Institution of Mechanical Engineers, Part H: Journal of Engineering in Medicine 225.11 (2011): 1050-1060.
3406, 3406-11, 3406-5, 3406-17, 3406-12 and 30 PCF
Gluek C, Zdero R, Quenneville CE. Evaluating the mechanical response of novel synthetic femurs for representing osteoporotic bone [published online ahead of print, 2020 Aug 28]. J Biomech. 2020;111:110018. doi:10.1016/j.jbiomech.2020.110018
3503
Lamb, Jonathan N et al. Comparison of axial-rotational postoperative periprosthetic fracture of the femur in composite osteoporotic femur versus human cadaveric specimens: A validation study. Proceedings of the Institution of Mechanical Engineers. Part H, Journal of engineering in medicine vol. 236,7 (2022): 973-978. doi:10.1177/09544119221092842
3503
Tibia
Heiner, A.D., Structural properties of fourth-generation composite femurs and tibias. J Biomech, 2008. 41(15): p. 3282-4.
3403, 3401
Gardner, M.P., et al., Mechanical evaluation of large-size fourth-generation composite femur and tibia models. Ann Biomed Eng, 2010. 38(3): p. 613-20.
3406, 3402
Humerus
Dunlap, J.T., et al., Structural properties of a novel design of composite analogue humeri models. Ann Biomed Eng, 2008. 36(11): p. 1922-6.
3404
Aziz, MSR, et al. Biomechanical measurement of stopping and stripping torques during screw insertion in five types of human and artificial humeri. Proc Inst Mech Eng [H] J. Engineering in Medicine. 2014. 228 p. 446 – 455.
3404
Aziz MSR, et al. Biomechanical Measurements of Stiffness and Strength for Five Types of Whole Human and Artificial Humeri. Journal of Biomechanical Engineering, 2014, 136(5), 051006-1-10
3404
Aziz MSR, et al. Biomechanical Measurements of Cortical Screw Purchase in Five Types of Human and Artificial Humeri. Journal of the Mechanical Behavior of Biomedical Materials, 2014, 30, 159-167
3404
Biomechanical Spine
Sherrill JT, Siddicky SF, Davis WD, Chen C, Bumpass DB, Mannen EM. Validation of a custom spine biomechanics simulator: A case for standardization.
J Biomech. 2020 Jan 2;98:109470. doi: 10.1016/j.jbiomech.2019.109470. Epub 2019 Nov 6. PMID: 31740014; PMCID: PMC6952059.
3430-25
Camisa W, Leasure J, Buckley J. Validation of a synthetic lumbar spine. The Spine Journal. 29th Annual NASS meeting. 2014.
3430-25
Wang T, Ball JR, Pelletier MH, Walsh WR. Biomechanical evaluation of a biomimetic spinal construct. Journal of experimental orthopaedics. 2014, 1:3.
3430-34
Wang T, Ball JR, Pelletier MH, Walsh WR. Initial experience with synthetic spinal motion segments: biomechanical assessment of high cycle and implant performance. Orthopaedic Research Society 2014. Poster presentation 0715.
3430-34
Wang T, Pelletier MH, Walsh WR. Evaluation of a synthetic functional spine unit: pure moment cycle test. Spine society of Australia. 2013, 5.3.
3430-34
Campbell JR, Imsdahl SI, Ching RP. Evaluation of a synthetic L2-L5 spine model for biomechanical testing. Canadian Society of Biomechanics 2012. Poster presentation.
3430-25
Campbell JR, Imsdahl SI, Ching RP. Evaluation of a synthetic functional spine unit. Northwest biomechanics symposium. 2011.
3430-34
Domann, John et al. The Analogue Spine Model: The First Anatomically and Mechanically Correct Synthetic Physical Model of the Lumbar Spine. The Spine Journal, Volume 11, Issue 10, S155 - S156. 2011.
3430-34
Review
Elfar, John et al. Composite Bone Models in Orthopaedic Surgery Research and Education. J AAOS. 22.2 (2014): 111–120. PMC. Web. 4 Nov. 2016
Zdero R, Brzozowski P, Schemitsch E. Biomechanical properties of artificial bones made by Sawbones: A review. Medical Engineering and Physics 118 (2023) 104017.